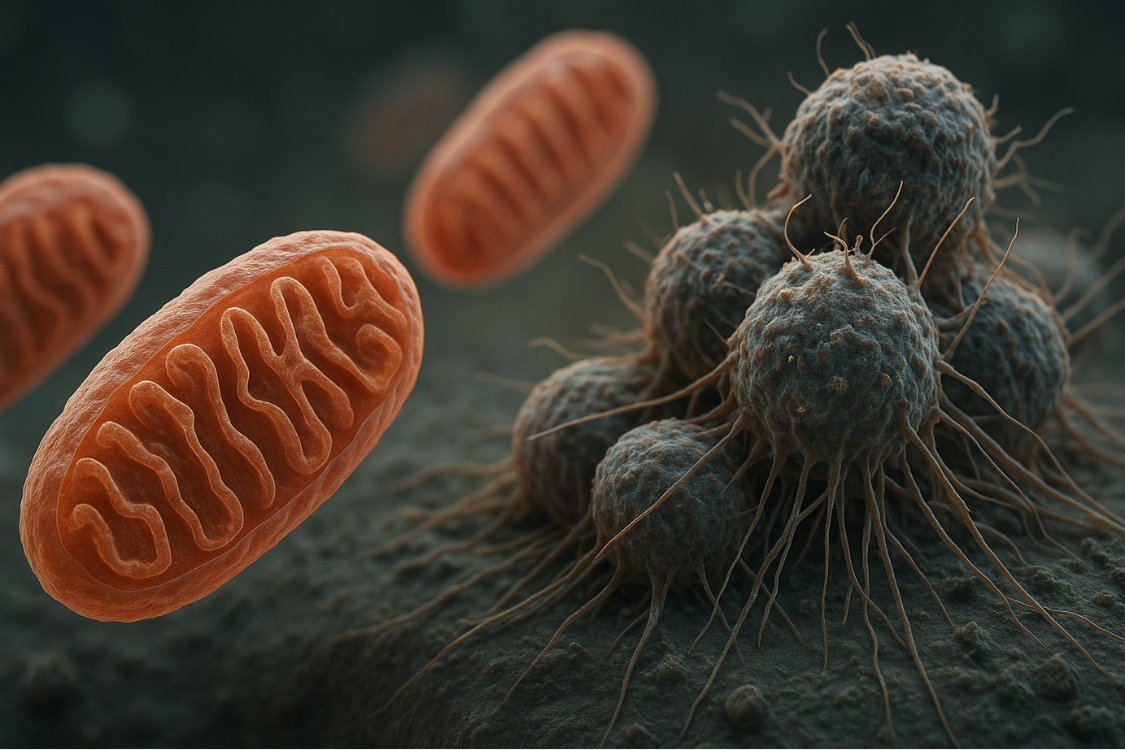
Mitochondria and Cancer: How These Powerhouses Shape Tumor Growth and Treatment
|
|
Time to read 8 min
100% Money Back Guarantee
|
|
Time to read 8 min
Can tiny parts inside your cells influence how cancer grows or responds to treatment?
Scientists now know that mitochondria, often called the cell’s powerhouse, do much more than just make energy. In cancer cells, these organelles shift their normal roles, change how energy is made, and even help tumors grow and resist therapy. As research into cancer metabolism expands, more attention is being given to mitochondrial function, mitochondrial DNA mutations, and mitochondrial reactive oxygen species.
This article explains how mitochondria affect cancer cell survival and cancer metabolism and how they are becoming a key target in cancer treatment.
Mitochondria are small parts inside your cells that do much more than just help you stay energized. These structures are essential for cell metabolism, helping cells grow, communicate, and even decide when to die.
Mitochondria turn nutrients like glucose and fatty acids into energy through a process called oxidative phosphorylation. This happens along the electron transport chain in the mitochondrial inner membrane and creates ATP (adenosine triphosphate), the main energy source for eukaryotic cells. This process also involves oxygen and is more efficient than other energy systems, like aerobic glycolysis.
Besides making energy, mitochondria play several other roles that help keep cells balanced and healthy. These functions are especially important in cancer biology, where they are often disrupted.
Cell death (apoptosis): Mitochondria help decide when damaged or dangerous cells should self-destruct.
Reactive oxygen species (ROS) control: They manage mitochondrial ROS production, which affects both cell signaling and oxidative stress.
Calcium balance: Mitochondria regulate calcium levels, which support proper cell function and communication.
Gene transcription: Signals from mitochondria influence how certain genes turn on or off.
Signal transduction pathways: They help modulate pathways that control cell survival, growth, and inflammation.
Mitochondria in cancer do more than fuel tumor cells—they reshape energy systems and influence cancer progression. Changes in mitochondrial function, structure, and communication give cancer cells the tools they need to grow, survive, and resist stress.
"Changes in mitochondrial function, structure, and communication give cancer cells the tools they need to grow, survive, and resist stress.”
Cancer cells often shift away from oxidative phosphorylation and rely on aerobic glycolysis, a hallmark known as the Warburg Effect. Even with oxygen present, this rapid energy-making process supports fast cell division, boosts mitochondrial biogenesis, and helps tumors adapt to harsh environments.
Studies published in Nature Communications and Nature Reviews Cancer highlight that metabolic rewiring is a common feature across multiple cancers, including breast and lung cancers. This reprogramming supports tumor growth, metastasis, and therapy resistance.
This switch allows tumor cells to generate energy quickly and avoid programmed cell death. It also supports glutamine metabolism and fatty acid breakdown, both of which are important for DNA repair and continued cell survival. Altered glucose metabolism further enhances the ability of tumor cells to outgrow normal tissue and spread.
Mitochondrial dysfunction disrupts normal cellular metabolism and contributes to cancer development. Damaged mitochondrial DNA (mtDNA) or mutations in the mitochondrial genome can reduce mitochondrial respiration and increase oxidative stress. These changes in mitochondrial biology allow cancer cells to survive in low-oxygen conditions and encourage abnormal cell signaling, aiding in cancer progression and treatment resistance.
Mutations in the mitochondrial genome are increasingly linked to cancer risk and tumor behavior. These mutations affect how energy is made, how cells communicate, and how the body responds to damage.
Somatic mtDNA mutations interfere with energy production and oxidative phosphorylation. This leads to reduced cell death, uncontrolled cancer cell proliferation, and greater tumor growth. Such mutations have been identified in multiple cancers, including breast cancer patients, bladder cancer, and renal cell carcinoma.
Some mitochondrial enzyme mutations, such as those in SDH (succinate dehydrogenase), are associated with the production of oncometabolites that may disrupt normal cell function. These changes modulate signal transduction pathways and protein kinase activity, driving uncontrolled growth. Oncometabolites can also interfere with gene regulation by blocking transcription factors and affecting mitochondrial retrograde signaling.
When mitochondrial function is impaired, stress signals are sent back to the nucleus—a process called mitochondrial retrograde signaling. This alters gene expression and activates transcription factors like hypoxia-inducible factor (HIF), leading to increased blood vessel formation and cancer cell migration. This process is common in aggressive cancers and further links mitochondrial dysfunction to cancer progression.
Mitochondria in cancer also influence how healthy cells behave. Tumors can change their environment by manipulating nearby stromal cells, turning them into energy suppliers.
Tumor cells send signals that change how nearby stromal and immune cells process energy. These supportive cells break down fatty acids and glucose, then release nutrients like lactate, which feed into the cancer cell’s mitochondrial metabolism. This interaction supports continued tumor growth and highlights the broader role of mitochondria in cancer.
Lactate, once thought to be waste, is now seen as fuel for tumor cells through mitochondrial respiration. This process promotes cancer cell proliferation and is especially active in cancer stem cells. Advanced imaging techniques like positron emission tomography (PET) are helping researchers better understand these shifts in cellular metabolism.
Emerging studies suggest that in rare cases, cancer cells may acquire mitochondria from nearby cells, which could potentially support survival under stress. This process may play a key role in cancer progression and resistance to treatment.
Tumor cells can physically hijack mitochondria from nearby immune cells and stromal cells through vesicle transport or direct contact. These borrowed organelles restore mitochondrial metabolism and support ATP production, especially under stress or low-oxygen conditions. This is critical for tumor cells in environments with poor mitochondrial function or damaged mitochondrial DNA (mtDNA mutations).
Emerging research suggests that mitochondrial transfer may play a role in how some tumor cells resist chemotherapy or radiation, though this idea is still being investigated. Transferred mitochondria could help restore energy production in damaged cancer cells, potentially stabilizing their metabolism and supporting mitochondrial biogenesis. This may improve mitochondrial function and help cancer cells survive treatment. In cancers such as lung cancer and renal cell carcinoma, the long-term effects of mitochondrial transfer on nearby healthy cells remain unclear and are still being studied.
Mitochondria in cancer cells do more than produce energy—they also help tumors evade therapy. Their shifting roles in cancer metabolism and cell signaling influence how treatments work.
Damaged mitochondria or abnormal mitochondrial genome activity can help cancer cells avoid programmed cell death. This weakens the impact of chemotherapy by altering oxidative phosphorylation and glutamine metabolism. Mitochondrial dysfunction is especially common in aggressive cancers like breast cancer and bladder cancer.
To stay alive, cancer cells trigger mitophagy to clear out faulty mitochondria. This limits oxidative stress and mitochondrial ROS production. As a result, tumors maintain energy through adapted glucose metabolism and continue growing despite treatment.
Researchers are exploring new ways to target mitochondrial biology and energy systems. Therapies that block enzymes like succinate dehydrogenase or modulate mitochondrial retrograde signaling may reduce tumor growth and improve cancer treatment. Combining these approaches with standard care could help overcome resistance, especially in breast cancer patients and those with pathogenic mutations tied to mitochondrial function.
Therapies that target mitochondria are gaining interest among researchers. These approaches show potential, especially in cancers with high mitochondrial activity.
Experimental drugs, such as IDH1/2 (isocitrate dehydrogenase 1 and 2) inhibitors and glutaminase blockers, are being investigated for their effects on mitochondrial energy metabolism. These experimental therapies aim to disrupt glucose metabolism and mitochondrial energy pathways in cancer cells, which may impact their survival.
Researchers are exploring how combining these drugs with standard treatments may reduce resistance. Positron emission tomography (PET) imaging also helps track changes in cell metabolism, improving how doctors measure treatment success.
Mitochondria and cancer are more connected than many once believed. These organelles are not only central to energy production but also play key roles in cancer cell survival, oxidative stress, and treatment resistance. From mitochondrial DNA mutations to changes in cellular metabolism and mitochondrial dysfunction, their impact reaches nearly every part of cancer biology. As researchers uncover how mitochondrial metabolism, reactive oxygen species, and mitochondrial proteins shape tumor growth, new treatment options are emerging. A deeper understanding of mitochondrial function may help inform the development of safer, more targeted cancer therapies.
Mitochondria in cancer cells control energy metabolism, cell survival, and tumor growth through altered mitochondrial function and ROS production.
Yes, mitochondrial DNA mutations have been linked to changes in energy production and cell behavior that may contribute to cancer progression.
The Warburg effect is when cancer cells prefer to make energy through glucose fermentation even when oxygen is available, fueling rapid growth.
Cancer cells change their metabolism to produce energy quickly, avoid cell death, and support continuous cell proliferation and growth.
Researchers are exploring the role of mitochondrial metabolism in cancer treatment and resistance mechanisms.
Doblado, L., Lueck, C., Rey, C., Samhan-Arias, A. K., Prieto, I., Stacchiotti, A., & Monsalve, M. (2021). Mitophagy in Human Diseases. International journal of molecular sciences, 22(8), 3903. https://doi.org/10.3390/ijms22083903
Khan, J., Bareja, C., Dwivedi, K., et al. (2025). Identification and validation of a metabolic-related gene risk model predicting the prognosis of lung, colon, and breast cancers. Scientific Reports, 15, 1374. https://doi.org/10.1038/s41598-025-85366-8
Potter, M., Newport, E., & Morten, K. J. (2016). The Warburg effect: 80 years on. Biochemical Society transactions, 44(5), 1499–1505. https://doi.org/10.1042/BST20160094
Schubert, A. D., Channah Broner, E., Agrawal, N., London, N., Pearson, A., Gupta, A., Wali, N., Seiwert, T. Y., Wheelan, S., Lingen, M., Macleod, K., Allen, H., Chatterjee, A., Vassiliki, S., Gaykalova, D., Hoque, M. O., Sidransky, D., Suresh, K., & Izumchenko, E. (2020). Somatic mitochondrial mutation discovery using ultra-deep sequencing of the mitochondrial genome reveals spatial tumor heterogeneity in head and neck squamous cell carcinoma. Cancer letters, 471, 49–60. https://doi.org/10.1016/j.canlet.2019.12.006
Wilson D. F. (2017). Oxidative phosphorylation: regulation and role in cellular and tissue metabolism. The Journal of physiology, 595(23), 7023–7038. https://doi.org/10.1113/JP273839