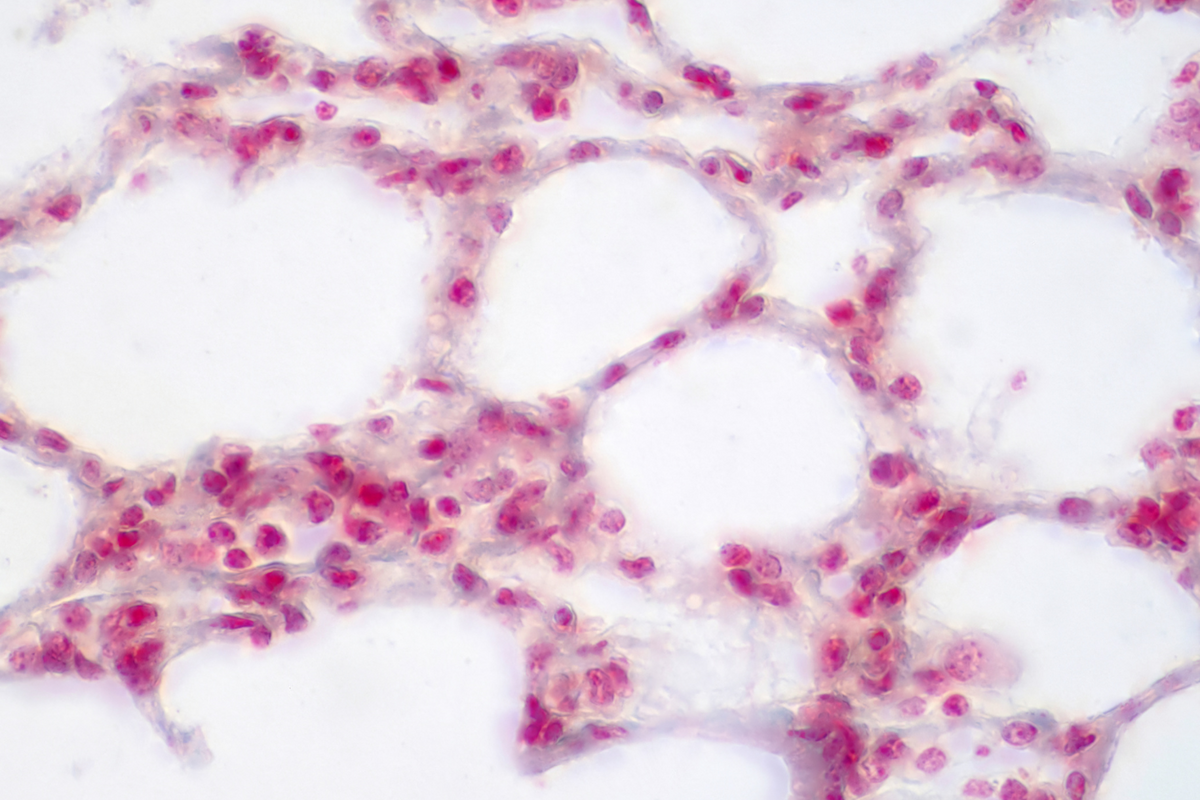
How Do Mitochondria Make Energy? | Understanding Cellular Powerhouses
|
|
Time to read 8 min
100% Money Back Guarantee
|
|
Time to read 8 min
Mitochondria are essential organelles in eukaryotic cells that play a central role in energy production and cellular metabolism. Often called the "powerhouses of the cell," mitochondria convert chemical energy from nutrients into adenosine triphosphate (ATP), the energy currency required for physiological processes.
Through cellular respiration, including the citric acid cycle and oxidative phosphorylation, mitochondria produce ATP and support critical functions like cell signaling, programmed cell death, and cellular metabolism. The inner mitochondrial membrane, mitochondrial matrix, and mitochondrial proteins work together in these processes, highlighting the importance of mitochondrial activity for sustaining life in human cells.
Cellular respiration is a vital process in eukaryotic cells that converts chemical energy from nutrients into adenosine triphosphate (ATP), the primary energy currency used for physiological processes like cell signaling, division, and metabolism. This energy production pathway is essential for supporting activities in energy-intensive cells, such as muscle cells, nerve cells, and heart muscle cells.
This multi-step process includes glycolysis in the cytoplasm, followed by the citric acid cycle and oxidative phosphorylation in the mitochondria. Each stage is crucial, with glycolysis producing pyruvate and small amounts of ATP, while the citric acid cycle generates electron carriers like NADH and FADH2. Finally, oxidative phosphorylation in the inner mitochondrial membrane produces most of the cell's ATP, powering essential cellular functions.
Glycolysis is the first step in cellular respiration, where glucose is broken down into smaller molecules to release energy. This process occurs in the cytoplasm of eukaryotic cells and does not require oxygen, making it vital for aerobic and anaerobic respiration.
The main goal of glycolysis is to break one glucose molecule into two pyruvate molecules while producing small amounts of ATP and NADH. These products are then used in later stages of cellular respiration, ensuring a continuous supply of energy.
"Cellular respiration is a vital process in eukaryotic cells that converts chemical energy from nutrients into adenosine triphosphate (ATP).”
During glycolysis, glucose is broken down into two molecules of pyruvate through a series of enzymatic reactions. This process begins with glucose phosphorylation, where ATP is used to add a phosphate group, making glucose more reactive. The six-carbon sugar is then split into two three-carbon molecules.
As glycolysis progresses, the three-carbon molecules are further modified, resulting in the production of ATP and NADH. A total of two ATP molecules and two NADH molecules are produced per glucose molecule, contributing to energy metabolism in cells.
During intense exercise, glycolysis is critical for energy production, especially in cells with limited oxygen supply, such as muscle cells. It provides a rapid source of ATP to fuel essential physiological processes.
This process is also a key part of anaerobic respiration, which allows cells to generate energy without oxygen. For example, in certain cell types like cancer cells and red blood cells, glycolysis plays a dominant role in energy metabolism.
The citric acid cycle, also known as the Krebs cycle, is a series of chemical reactions that occur in the mitochondrial matrix. It is the second stage of cellular respiration and is responsible for oxidizing acetyl-CoA to produce energy-storing molecules such as NADH, FADH2, and ATP. The cycle also releases carbon dioxide as a byproduct. This process is essential for maintaining energy metabolism in cells.
Before entering the cycle, pyruvate from glycolysis is converted to acetyl-CoA in the mitochondrial matrix. Acetyl-CoA serves as the starting molecule for the citric acid cycle.
Production of NADH: Throughout the cycle, electrons are transferred to NAD+, forming three molecules of NADH per cycle.
Production of FADH2: One molecule of FADH2 is generated during the oxidation of specific intermediates.
Production of ATP: A single molecule of GTP (or ATP, depending on the cell type) is synthesized directly during the cycle.
The citric acid cycle is crucial for metabolizing acetyl-CoA and extracting high-energy electrons for ATP synthesis. It plays a central role in breaking down carbohydrates, fats, and proteins into usable energy.
This cycle connects to other metabolic pathways, such as fatty acid oxidation and amino acid metabolism, ensuring that cells efficiently use different energy sources. It also provides intermediates for biosynthetic pathways, supporting cell growth and division.
The electron transport chain (ETC) is the final stage of cellular respiration and occurs within the inner mitochondrial membrane. This series of protein complexes and electron carriers transfer electrons from NADH and FADH2 to oxygen, driving the production of ATP. The process also generates a proton gradient across the mitochondrial inner membrane, which powers ATP synthesis through ATP synthase.
The electron transport chain consists of several coordinated steps that produce ATP:
Transfer of Electrons: Electrons from NADH and FADH2 are passed to protein complexes in the ETC, releasing energy as they move down the chain.
Proton Gradient Formation: Energy from electron transfer pumps protons from the mitochondrial matrix to the intermembrane space, creating an electrochemical gradient.
ATP Synthase Activation: Protons flow back into the mitochondrial matrix through ATP synthase, a protein complex that uses this movement to synthesize ATP from ADP and inorganic phosphate.
The ETC is vital for generating most of the ATP produced in cellular respiration. Depending on cellular conditions and efficiency, the process can make approximately 28–32 ATP molecules per glucose molecule.
This chain is also essential for oxygen consumption, as oxygen acts as the final electron acceptor, forming water as a byproduct. The ETC cannot function without oxygen, disrupting energy metabolism and cellular processes.
Oxidative phosphorylation combines the ETC and ATP synthesis to produce large amounts of ATP. Electrons transferred through the ETC create a proton gradient, which powers ATP synthase to convert ADP to ATP. This process, occurring in the mitochondrial inner membrane, is critical for energy production in eukaryotic cells.
ATP synthase is a protein complex that uses the energy of the proton gradient to catalyze ATP production. As protons flow through ATP synthase, the protein rotates, enabling the enzymatic conversion of ADP and inorganic phosphate into ATP.
This mechanism ensures a constant supply of ATP, which powers cellular activities like muscle contraction, nerve signaling, and cell division. The efficiency of ATP production is central to maintaining healthy cellular metabolism.
Genetic, environmental, and lifestyle factors can influence mitochondrial energy production. Understanding these factors can help optimize mitochondrial function and overall cellular health.
Mitochondrial DNA, inherited maternally, is critical in encoding proteins essential for mitochondrial function. Mutations in mitochondrial DNA can disrupt the electron transport chain and oxidative phosphorylation, leading to mitochondrial diseases and reduced ATP production.
Mutations in nuclear genes that encode mitochondrial proteins can impact mitochondrial function and have been associated with certain diseases, including neurodegenerative disorders.
Toxins such as heavy metals and pesticides can damage mitochondria by increasing the production of reactive oxygen species (ROS), impairing ETC and ATP synthesis.
Nutrient availability also influences mitochondrial activity. A lack of essential nutrients like vitamins and minerals disrupt processes like fatty acid oxidation and the citric acid cycle, reducing energy production.
Diet and exercise directly affect mitochondrial health. Consuming nutrient-rich foods supports mitochondrial biogenesis and ATP production, while regular exercise enhances mitochondrial efficiency and energy metabolism.
Chronic stress may increase ROS levels and affect mitochondrial function. Relaxation techniques could help reduce stress and support overall cellular health.
Supporting mitochondrial health involves a combination of proper nutrition, physical activity, and minimizing oxidative stress to optimize energy production.
Nutrients like coenzyme Q10, B vitamins, and magnesium contribute to mitochondrial processes like ATP synthesis and energy production. Consuming foods like leafy greens, fatty fish, and nuts may support mitochondrial function and energy metabolism.
Regular physical activity improves mitochondrial function and biogenesis. Beneficial exercises include:
Aerobic Exercise: Activities like jogging or swimming improve oxygen use and energy production.
Strength Training: Builds muscle and enhances mitochondrial efficiency.
High-Intensity Interval Training (HIIT): Boosts mitochondrial biogenesis and ATP production.
Antioxidants can help reduce ROS levels, potentially supporting mitochondrial health. Key antioxidants include vitamins C and E, selenium, and glutathione.
Adopting techniques like stress management, proper sleep, and reducing exposure to toxins minimizes oxidative stress, ensuring mitochondria function optimally for energy production.
Mitochondria are vital for energy production, fueling essential cellular processes like metabolism, cell signaling, and ATP synthesis. From glycolysis and the citric acid cycle to the electron transport chain and oxidative phosphorylation, mitochondria play a central role in maintaining cellular health.
Factors like genetics, lifestyle, and nutrition influence mitochondrial function, making it important to support these powerhouses through a healthy diet, regular exercise, and stress management. Optimizing mitochondrial health ensures sustained energy and improved overall well-being.
During oxidative phosphorylation, the most efficient stage of cellular respiration, mitochondria produce about 34 ATP molecules per glucose molecule.
Yes, regular exercise, a nutrient-rich diet, and managing oxidative stress can enhance mitochondrial energy production and efficiency.
Signs include fatigue, muscle weakness, neurological issues, and problems with energy-intensive organs like the heart and brain.
Mitochondria convert chemical energy from nutrients into ATP, providing the energy cells need to perform critical functions.
Nutrients like B vitamins, coenzyme Q10, magnesium, and antioxidants help maintain mitochondrial function and energy production.
Alabduladhem TO, Bordoni B. Physiology, Krebs Cycle. [Updated 2022 Nov 23]. In: StatPearls [Internet]. Treasure Island (FL): StatPearls Publishing; 2025 Jan-. Available from: https://www.ncbi.nlm.nih.gov/books/NBK556032/
Alberts B, Johnson A, Lewis J, et al. Molecular Biology of the Cell. 4th edition. New York: Garland Science; 2002. The Mitochondrion. Available from: https://www.ncbi.nlm.nih.gov/books/NBK26894/
Chandel N. S. (2021). Glycolysis. Cold Spring Harbor perspectives in biology, 13(5), a040535. https://doi.org/10.1101/cshperspect.a040535
Chaudhry R, Varacallo MA. Biochemistry, Glycolysis. [Updated 2023 Aug 8]. In: StatPearls [Internet]. Treasure Island (FL): StatPearls Publishing; 2025 Jan-. Available from: https://www.ncbi.nlm.nih.gov/books/NBK482303/
Kozlov, A. V., Javadov, S., & Sommer, N. (2024). Cellular ROS and Antioxidants: Physiological and Pathological Role. Antioxidants (Basel, Switzerland), 13(5), 602. https://doi.org/10.3390/antiox13050602